Particle Physics and Cosmology Group
Max Planck Institute for Physics, Munich

Quantum Chromodynamics at high energies
My research interests are in Quantum Chromodynamics (QCD) with a particular focus on the theory at high energies or small Bjorken-x. As evidenced by experiments at the HERA electron-proton collider at DESY (see figure on the right), the physics of this high energy regime is primarily dominated by rapidly growing low momentum self-interacting gluons that abound in density in the hadron wavefunction. The stability of the theory against such endless gluon proliferation requires gluon recombination and screening effects to bring about a "saturation" in the phase space occupancy of gluon modes at n>>1. An occupation number greater than 1 is considered the hallmark of a high density system and the large mode occupancy at saturation suggests that QCD at high energies is remarkably classical. The coupling however is weak because of repulsion among the gluons who try to occupy higher momentum states with increase in energy and the characteristic momentum scale at which the occupancy becomes maximal is known as the saturation momentum scale Q (x). This emergent quantity grows with energy and at sufficiently high energies qualifies as a semi-hard scale that governs the running of the QCD coupling.

At small Bjorken-x, gluons dominate the hadron wavefunction.
Figure taken from arXiv: 1212.1701
QCD in this regime is therefore extremely interesting because highly non-perturbative phenomena involving strongly correlated gluonic matter can be systematically studied using perturbative techniques. Nuclear physics becomes simple but the full richness and complexity of QCD as contained in the gluon self-interactions is preserved in this regime. The theoretical framework that describes the dynamics of gluon saturation in QCD is a classical, stochastic effective field theory (EFT) known as the Color Glass Condensate (CGC). The CGC is also used synonymously to denote the gluon saturated novel matter that is conjectured to emerge at high energies in hadrons and nuclei. It is different from a Bose-Einstein condensate in the sense that the small x gluons are not peaked in density around zero momentum but at the saturation momentum scale.
​
​
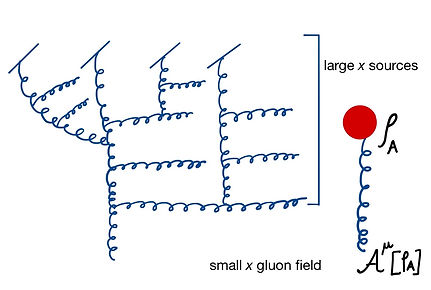
The essence of the CGC is a separation of the degrees of freedom inside a high energy hadron into time dilated large momentum partons that play the role of static color sources generating dynamical low momentum gluon fields that behave almost classically. This temporal or longitudinal momentum separation is at the heart of the "glass" appellation. The large x sources which possess color charge are randomly distributed and live much longer than characteristic time scales of the interaction with external "projectiles" used to probe the hadron or nucleus in scattering experiments. A knowledge of the probability distribution of these color sources completely specifies the EFT. This gauge-invariant, non-perturbative object is a functional weight function of the color charge density of the large x sources and is analogous to parton distribution functions (PDFs) but contains much more information about the n-body correlations among gluons. The arbitrariness of the scale separating the static sources from dynamical fields is exploited to derive a renormalization group equation (RGE) for this weight function known as the Jalilian-Marian, Iancu, McLerran, Weigert, Leonidov, Kovner (JIMWLK) equation. This is generated in a self-similar pattern wherein the contributions from gluon field modes at higher energies are integrated out and absorbed into the source charge densities at the evolved scale.
Non-linear evolution in the CGC EFT. As one evolves towards smaller x, classical gluon fields at a certain small x<x' qualify as quantum fluctuations that act as sources generating small x gluons for the next step of evolution.
s
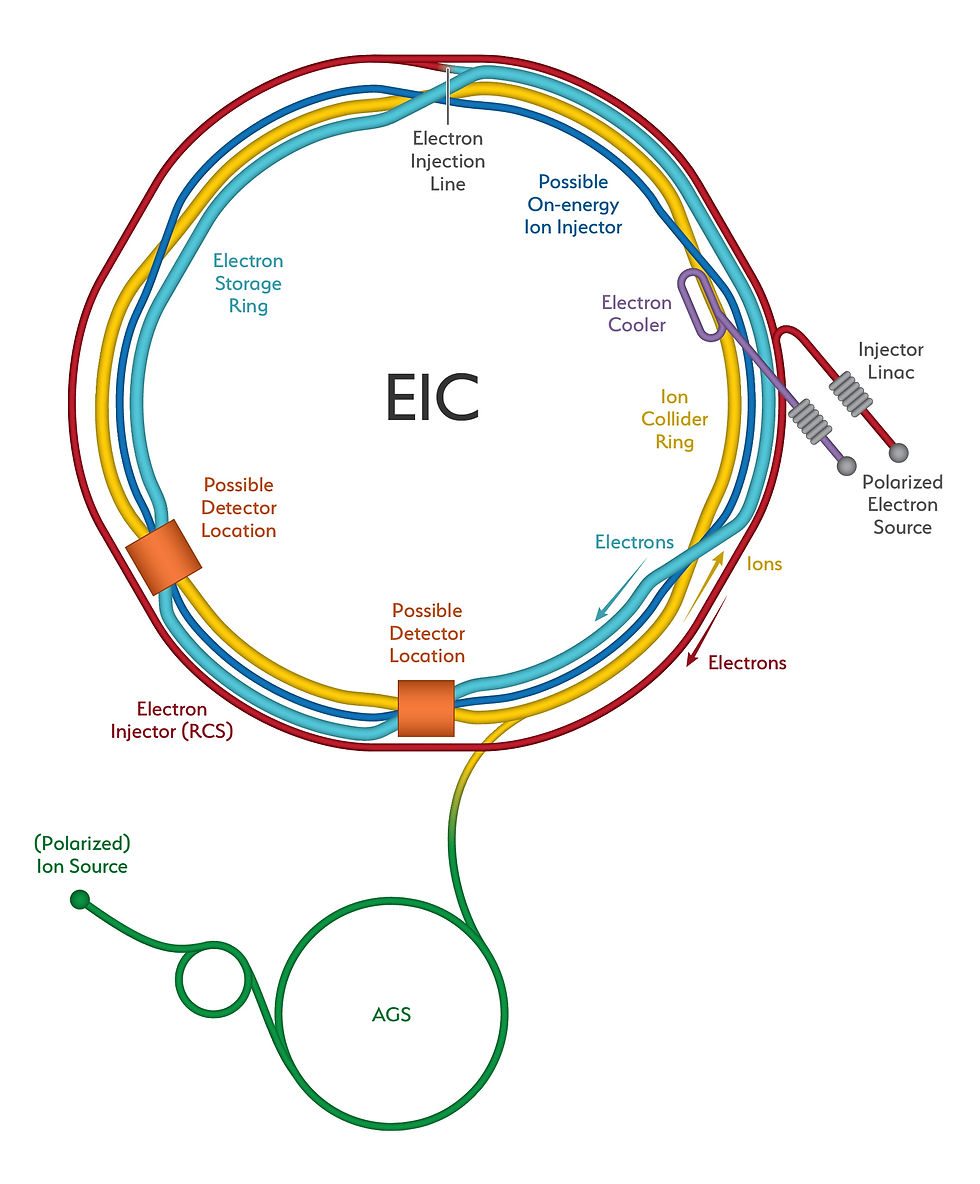
Probing gluon saturation with precision at an Electron-Ion Collider
One of the principal objectives of my doctoral work, done in collaboration with my advisor Raju Venugopalan, is to use the CGC EFT to perform precision theory computations for experimentally measurable processes that hold the potential of providing access to the uncharted regime of universal, saturated gluonic matter. This is an important endeavor because it complements the outstanding precision of current and forthcoming experimental data and helps draw meaningful conclusions or make conclusive predictions about the same.
​
2
Towards this end we have performed the first CGC computations of the differential cross-section for the inclusive production of a prompt photon in conjunction with a quark-antiquark dijet in nuclear deeply inelastic scattering (DIS) in the high energy "Regge-Gribov" kinematics of small Bjorken-x at fixed, large momentum resolution Q . This includes both the leading order and its next-to-leading order extension in the QCD coupling, in the CGC power counting. This computation is important and timely because of the prospect of such a measurement at a future high energy, high luminosity Electron-Ion Collider (EIC). The EIC which will be set up at the Brookhaven National Laboratory in Long Island, USA will be the world's most powerful electron microscope to study the emergent dynamics of quarks and gluons. It will distinguish itself from previous and existing high energy collider facilities by providing a clean environment to study the nuclear landscape and the capability to probe a wide range of phenomena by virtue of its unprecedented luminosities and high center-of-mass energies. Because nuclear targets are possible to collide with at the EIC, it offers a heightened possibility of accessing the gluon saturation regime than previous facilities such as the HERA, due to nuclear enhancement in the phase space density of small x gluons by approximately A , where A is the nuclear mass number.


One can visualize (see figures above on the right) the photon+dijet production at high energies as a fluctuation of the virtual photon exchanged in the electron scattering into a quark-antiquark dipole which interacts with the gluon dominated nuclear matter via electric/color charges and emits a real photon either before or after this interaction. The information about the color charge interaction is embedded in gauge invariant correlators of path ordered exponentials or Wilson lines which is then translated into the distribution of saturated gluons. These arise in the theoretical computation in the momentum space Feynman rules for the quark and gluon propagators in the background of the strong classical field of small x gluons.
Although quite tedious and non-trivial in terms of analytical evaluation, the results for photon+dijet production process at small x are a treasure trove of useful information. A number of first CGC results can be obtained in certain limits of our results. These include the first results for the cross-section for inclusive dijet production, at next-to-leading order in the QCD coupling, in the limit of soft final state photons and similar results for other multiparticle measurements such as inclusive photon and photon plus jet production. The latter channel was recently studied in depth for the first time at the kinematical ranges of the Brookhaven EIC. This work was performed in collaboration with researchers at BNL namely Björn Schenke and Farid Salazar, in addition to Raju. We observed a systematic suppression and broadening of the back-to-back peak in the azimuthal angle distribution of the associated yield of photons with a jet trigger. This is a well-known consequence of multiple scattering effects associated with high gluon densities that signal the onset of saturation. While such phenomenology was done previously for electron-hadron(nucleus) scattering for the case of dihadrons, our analysis for photon-jet angular correlations suggest that inclusive photon plus jet final states would be indeed interesting to explore at the EIC. The challenge remains, however, to reconstruct small k-t jets in order to access the saturation regime, because the small x constraint at the EIC center-of-mass energies severely restricts the transverse momenta ranges for final states particles in such differential measurements. This is a generic issue for inclusive differential measurements at the EIC and must be thought through in detail in order to avail the wealth of non-trivial information accessible in such processes.
High energy QCD and its connections with gravity
An intriguing idea that I am interested in exploring is the correspondence between the infrared structure of QCD and gravity. The classicalization of QCD at high energies brought in by gluon saturation provides avenues for investigating aspects of the infrared which are general for gauge theories, such as memory effects that share triangular equivalence to soft theorems and asymptotic symmetries. In addition there are also simplifications known in the literature that occur in multi-Regge kinematics for both gauge theory and gravity amplitudes. In the former case this is described by Lipatov's EFT which is formally equivalent to the CGC picture. This gives us all the more motivation to find parallels between these theories in the high energy limit. In theories with color, there is a symmetry known as the "color-kinematics duality" which allows us to obtain classical gravitational solutions as a "square'' of the solutions to classical Yang-Mills equations. I am eager to explore if this "classical double copy'' idea can be exploited to derive such a relationship for QCD in the high energy Regge limit. This is a completely new field of research for me but I am excited to pursue this endeavor through the course of my postdoctoral research. More on this as I learn some more...